We get a LOT of questions about volcanoes, including how to “know if she’ll blow.” There are a number of ways we can track magma movement at depth, including monitoring deformation and tracking “LP’s” – long-period (low-frequency) seismic tremors indicative of deep fluid movement. At late stages of unrest, we will start seeing “Vt’s” – short-period volcanic seismic evidence of rocks breaking – and often dramatic increases in Carbon-14-depleted Carbon Dioxide and Hydrogen Sulfide (burnt-match smell) gases. There is a good possibility that we can detect very early movement of magma at 30 – 40 km depths using Magnetotelluric systems, something I proposed many years ago, but there hasn’t been enough funding to try this. As I write this, edifice deformation still currently reaches out the longest time ahead of all these detection systems to give us warning of an impending eruption up to months ahead of time.
The term “deformation” is used by specialists in ground movement in the geosciences; these guys call themselves “geodesists”. Geodesists measure movement as a component of strain along an active fault, to try to get a sense of the elastic energy accumulating that could lead to an earthquake. Deformation is also used in volcanology to look for – and then track – inflation in a volcanic edifice. Deformation is measured in several ways:
1. Surveying the ground with high precision. This has been done at Yellowstone since the mid-1920’s, and those early data have helped us get a much better sense of how the huge caldera moves and breathes over time. It’s not at all unusual to find a section rising 20 cm (7.5 inches) in a few weeks. Typically, another part of the caldera will be deflating at the same time.
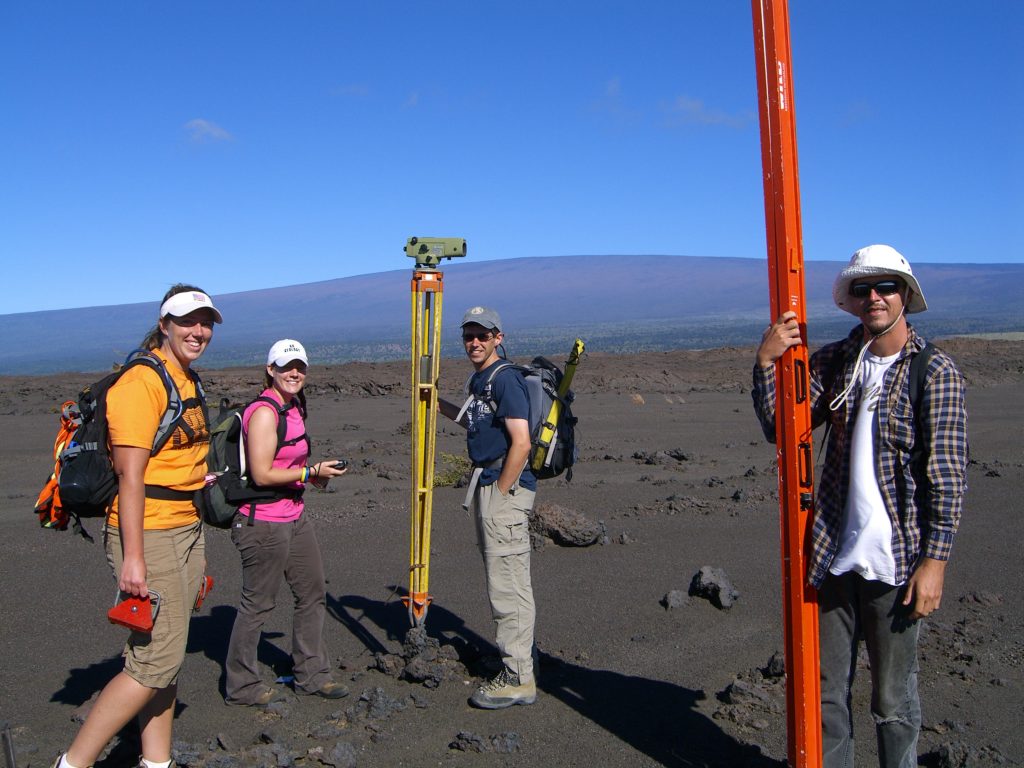
A geodetic survey team working on the Kilauea volcano southwest rift zone, Hawai’i.
2. Deploying tilt-meters. Originally these were long tubes of water laid out over the ground. If the ground under the flank of a volcano started tilting, a very tiny vertical movement would show up in amplified displacement of water in vertical tubes at the end of the long horizontal tube. Modern tiltmeters are ultra-sensitive cylinders placed in a vertical hole in the volcanic rock, then packed in with sand. The signal from these devices and all the following systems is generally telemetered back to a recording and monitoring station.
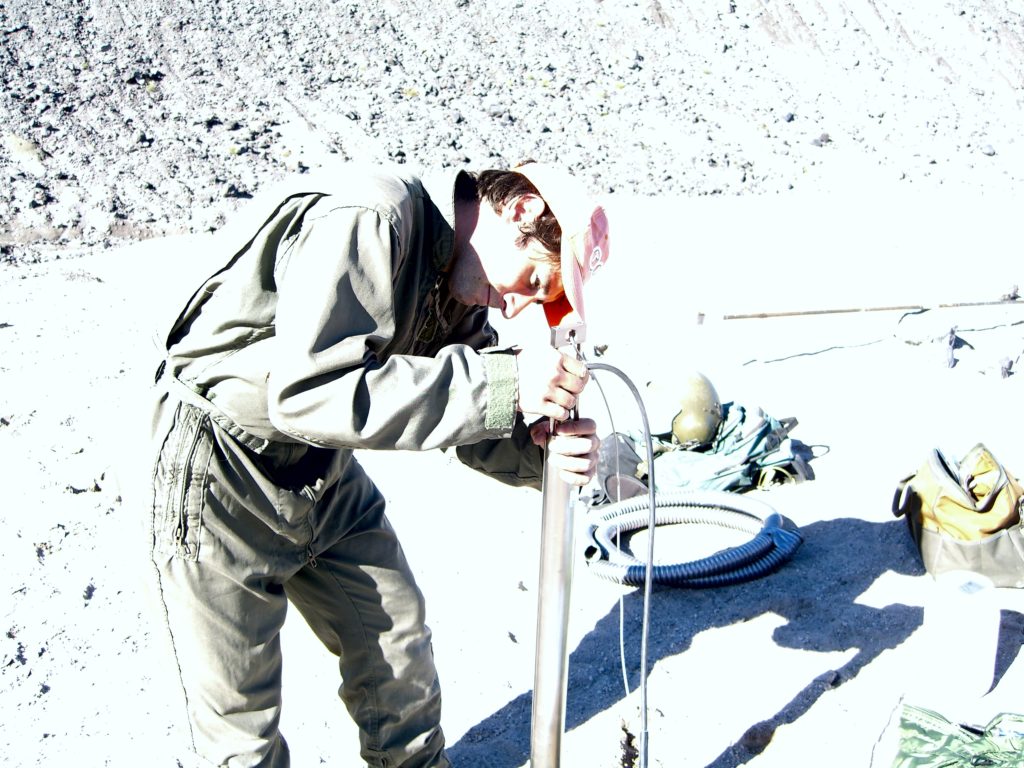
A modern tiltmeter being lowered into a borehole at Mount St Helens, WA.
3. Radar satellites – this approach is called InSAR (Interferometric Synthetic Aperture Radar). If two radar images can be captured over the same volcano, spaced days or weeks apart, they can be used to make interferograms. These look like colored Moiré patterns (see figures 47 and 48 below). They are generated with prodigious mathematical calculations to geometrically correct, and then ratio each individual pixel to another taken on a different satellite pass. These complex geometric corrections are sometimes called “rubber sheeting”, and the final result will show inflation over the surface of a volcano and its environs. Each rainbow-colored ring-set represents one radar wavelength (typically 5 – 15 centimeters) of uplift. These often form spectacular bulls-eyes centered over an inflating volcano or a deflating caldera, and I’ve seen several gorgeous examples at Ngiragongo volcano, in Central Africa; at Pavlof, Akutan, Okmok, Shishaldin, and many other volcanoes in the Aleutians (figure 47), as well as Mauna Loa (figure 48) and Kilauea volcanoes in Hawai’i.
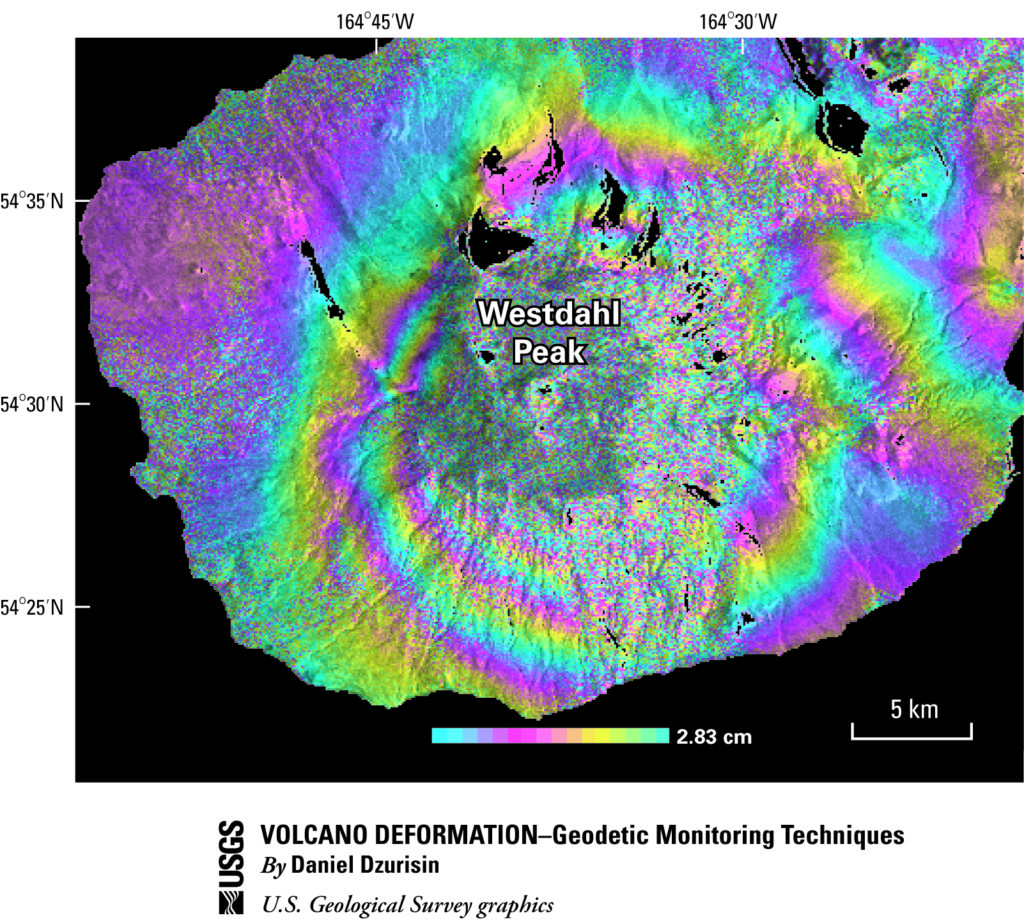
Westdahl volcano InSAR image, showing inflation between 1993 and 1998. Each color fringe means a net vertical change of 2.83 cm. While the volcano was inflating slowly, there was very low seismic activity. Image from Dan Dzurisin, USGS (Lu, Z., Wicks, C., Dzurisin, D., Thatcher, W., Freymueller, J.T., McNutt, S.R., and Mann, D. (2000b). Aseismic inflation of Westdahl Volcano, Alaska, revealed by satellite radar interferometry: Geophys. Res. Lett., 27, 1567–1570).
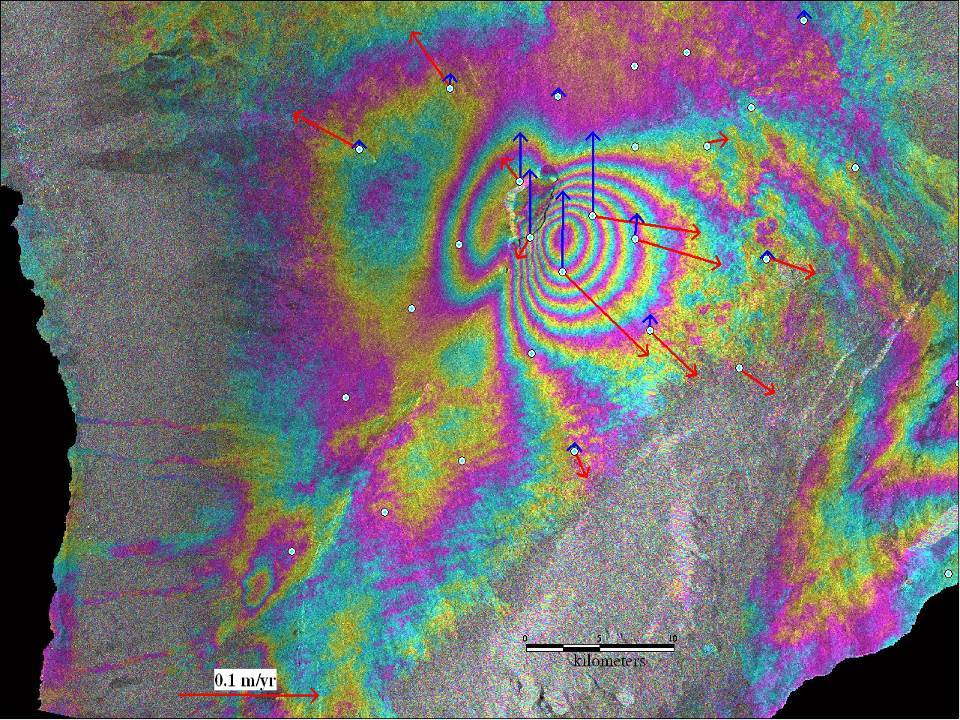
InSAR image of Mauna Loa volcano, Hawai’I, showing inflation fringes. Arrows show individual station sideways movement vectors from GPS as the volcano filled with new magma at relatively shallow depth. (image: US Geological Survey).
4. Gravity level-lines. This is like the precision survey leveling discussed earlier, but is done by making repeat measurements with three gravity meters over a line of stations every six months or so. It saves you having to traverse an extremely rugged volcanic field on foot to survey it (you can use a helicopter). All other things (including the water table) being equal, an inflating volcano will show up as a decrease in the gravity field – the station site that the gravimeter is sitting on is being moved upwards. If the station moves farther away from the Earth’s center, the pull of gravity falls off as 1/distance to the center of the Earth squared. For years I did this kind of survey every six months to monitor magma moving into the Harrat Rahat volcanic field, located east of Madinah al-Munawarrah (“Medina”) in Saudi Arabia. Seismic telemetry also showed small earthquakes associated with this magma movement into the Earth’s crust at the same time. The events died out before an eruption, and before I moved back to the United States, causing a lot of people to breathe a collective sigh of relief. This kind of on-again-off-again restive behavior is not at all unusual for a volcano. This same volcanic field erupted in 1256 AD and nearly wiped out the city of Medina at that time, so we take these things very seriously.
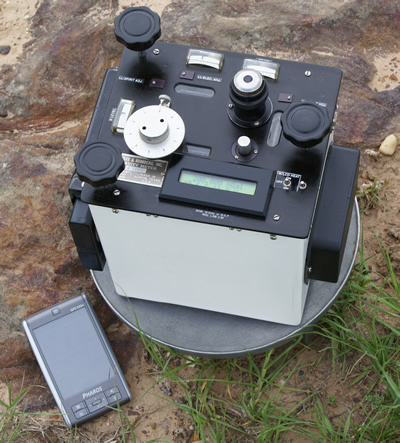
Typical gravimeter on a measurement base-plate (Image: LeCoste-Romberg Instruments).
5. Telemetered GPS. These use the same GPS satellites that you and I utilize in our cars or when hiking, but the precision measurements made by geodesists use different signals (called “p-code”) from the same satellites. This gives far higher precision when averaged for a fixed location and the data are stacked over a period of time. If two GPS stations on opposite sides of a volcano are moving apart… then something is filling the edifice in between (see figure 48).
6. We also instrument volcanoes with sensitive analog seismic sensors, and ultra-sensitive broadband seismic sensors. Some of these data are telemetered, some are recorded and just stored in the instrument box on a small hard-drive over the winter months until retrieved the following summer. That is, unless bears decide to play ball with one (which happens). One over-winter seismic network campaign at Katmai volcano in Alaska found 5 of 11 of our very expensive stations had been trashed by bears before the geodesists could get back to retrieve them.
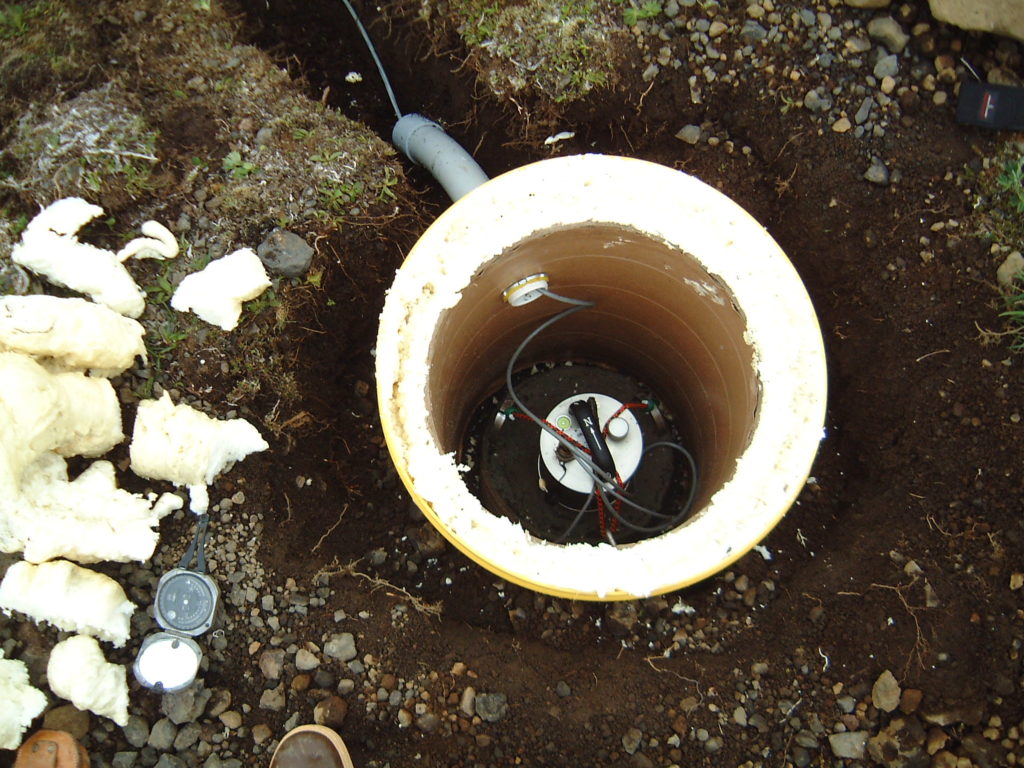
A ~$10,000 Guralp broadband seismometer the author installed in an underground vault on Akutan volcano, Aleutian Islands, Alaska. The Brunton compass in the lower left corner is used to orient the axes of the seismometer.
GPS is a fascinating field, and applies all through and far beyond the earth sciences. A brief run-down might be useful here.
The Global Positioning System was first envisioned by DARPA – the Defense Advanced Research Projects Agency of the Department of Defense – during the 1980’s. Navigation at that time was complex and difficult, and getting any sort of location precision over vast distances including oceans was very important to some people. Korean Air flight 007 was shot down by Russian warplanes in 1983 over what proved to be a small navigation error. Also, people targeting ballistic missiles would like to place them exactly on top of a hardened missile silo.
In the late 1980’s I was a young scientist working in the Venezuelan jungle, where our main form of navigation was to use 1:250,000-scale side-looking airborne radar (SLAR) maps. These were assembled by flight strips – radar images taken from high altitudes – and it was not unusual to find splice errors as large as 3 kilometers between strips. That means I could be standing on a single rock – and according to the SLAR map, half of the rock was 2 miles away from the other half of the rock. It gives the expression “huge strides” quite a different meaning. I have been on a helicopter traveling for an hour over trackless forest using a half-meter-sized, million-scale roadmap of the country (except there are no roads in the jungle) and crudely-penciled lines with the azimuth, and distance in flight-minutes to the target site that we wanted to visit. If that helicopter’s fuel line had a single bug in it, we would have dropped down into the trees. Even assuming we had survived such a crash (the incident statistics gave me a 50% chance of surviving this sort of crash), how would you call in a rescue helicopter? How in the world would you even tell rescuers where you were, when the wreck-site could not be seen beneath the jungle canopy?!? Your location and its accuracy become a life-and-death issue very quickly.
I first began using a GPS device in the early 1990’s in Saudi Arabia. In the northern reaches of the country there is a vast plain (the al-Jalamid Plain) that is dead flat for hundreds of kilometers in all directions. Some of our staff had accidentally strayed across the Iraqi border, because there is no way to know where the boundary line (arbitrarily drawn by the British almost a century earlier) actually was. The first GPS units were incredibly slow, the size of a Betty Crocker cookbook, and didn’t always work – but the idea fascinated me. With a radio, I could then tell people where I was within a hundred meters or so at that time; we could now find someone and perhaps save a life.
Since then, hand-held GPS devices have shriveled to coin size (I have one on my wrist, tracking my steps and bike-rides), and many have maps built in. You can program them, collect precise tracks of where you walked or flew… the list of bells and whistles goes on and on.
But how do they work? What actually is out (or up) there?
The American GPS constellation has at any given time about 24 active satellites and a few loitering backup spares, and each one transmits a very faint signal on two frequencies – a digital signal for hand-held device use and another digital carrier (the “p-code”) that is used for more precision location acquisition. I’m talking about centimeter-size precision here. Both GPS frequencies were originally encrypted by the US military. Because the satellites belonged to them, for a long time the signals were deliberately “fuzzed” – this was called Selective Availability, or SA for short. If you had the digital key and a certain type of book-sized device, you could get relatively precise locations – within 10’s of meters. But the US Department of Defense didn’t want someone else using those same signals to pop an artillery round on top of an American military outpost. Even to this day, if you try to use a receiver and go faster than a commercial airliner (as in: a ballistic missile) it won’t work. It has a built-in fail-safe.
In the meantime, the rest of the world has become incredibly dependent on the American GPS constellation. Aircraft you fly in now use them to navigate – even use them to land with in bad weather. I could never summarize adequately all the ways and places where GPS is used right now. As I write this, the Russians have their own satellite constellation called GLONASS, the European Union has one called GALILEO, the Chinese have the BeiDou Navigation Satellite System (BDS), and the Indians have the Indian Regional Navigation Satellite System (IRNSS) in orbit. In each case, these were launched to avoid dependence on the United States for anything that was mission-critical. Except creativity, perhaps.
If you are surveying – or trying to see if two points on the opposite sides of a volcano are moving apart from each other (uh-oh), then you need great precision. With modern GPS technology, this precision can now be better than a centimeter horizontally and 2-3 centimeters vertically. In part this difference in horizontal vs. vertical precision is because for horizontal solutions you can subtract the atmosphere effect from two different near-horizon satellites – and also triangulate better. For vertical elevations, you have only satellites in one direction (above you). You cannot detect a GPS signal from beneath your receiver because the signal would have to travel all the way through the Earth.
GPS signals all use similar frequencies, but the signals are encoded differently to separate the satellites. As implied earlier, signals from each satellite are encoded, so you can’t use one for a ballistic missile guidance system unless you own the codes. As I said, above a certain aircraft speed, GPS won’t work.
The Russians and Chinese certainly didn’t want to be dependent on some signal that the Americans could fuzz – or even turn off. So, despite their crushing economic difficulties, they turned the best Russian minds onto building their own GPS constellation. The GLONASS signal is not encoded, and the energy transmitted is greater, so the signal-to-noise ratio is 5 times or 15 db better. Because of this, the signal penetrates tree canopy, so I could now use it in the trackless jungle where I used to work. The GLONASS system also uses 3 different frequencies, so you can reduce ambiguities and better calculate the complex differential atmospheric corrections. The GALILEO, BDS, and IRNSS systems are similar.
These five GNSS (Global Navigation Satellite Systems) are so precise that they even routinely calculate and correct for relativistic effects (called “frame dragging”)! There are also huge atmosphere effects that must be compensated for – dense air masses here and differentially ionized layers there. GLONASS even works on new American and European hand-held devices when the GPS signals are poor due to a restricted view of the satellite constellation – if you’ve ever been in steep canyons in southern Utah or New York City, you know what I mean here. Not wanting to be left behind, the American version of GNSS (and the only one that should technically be called “GPS”) is being upgraded.
All five of these GNSS systems use L-band frequencies to resolve ambiguities and increase precision – and penetrate the ionosphere. What does L-band mean? Look at your personal car’s GPS antenna-fin, and the smallest dimension on it will give you an idea of the wavelength for L-band. That’s the size of your optimum-reception GPS antenna, by the way.
The navigation problem is more than just triangulation. Three satellites near the horizon would serve for this; two would give you two possible location solutions, and three would reduce this to only one possible solution. But there are four unknowns in this complicated system, since you must also measure how long the stretch of space and air that each signal must travel through. The precision of your timing thus becomes utterly critical, because the speed of light is so huge (~300,000 km/second), and hand-held GNSS devices cannot carry $100,000 maser clocks. Thus, you must use a 4th satellite to help solve for the 4th unknown: 3 for position, 1 for a clock reference for your receiver. This part is basic high-school matrix algebra that I learned as a sophomore.
There are a few more complications. Because of the 3D density variations of the Earth’s atmosphere, you can get even better precision if you also reference a ground station. This will give you even better differential distance calculations – to do good back-corrections for the changing satellite orbits, the complex and varying atmosphere, rain, and snow cover, etc. However, during the Tohoku earthquake in early 2011, all of Japan jerked eastward about 10 centimeters/4 inches. Geodesists couldn’t see the whole shift with really great precision – because all their reference stations also jerked eastward.
So back to the case in hand – how does this help volcanologists? As I wrote earlier, if two telemetered GNSS receivers are moving away from each other, and there is a volcano in between them (this has been happening for years now with Mauna Loa, the largest volcano on Earth), then you are being given a warning that something is moving into the volcano’s edifice – the space in between the stations.
In 1989 we didn’t have such a warning before Redoubt volcano in Cook Inlet of Alaska erupted. A KLM Boeing 747 flew right into the ash cloud – and lost all four engines in rapid succession. I’ve listened to a recording of the captain’s voice as she tried to guide her flight crew in Dutch and talk with flight control in Anchorage in English. Her voice rose steadily a full octave before she finally yelled “Anchorage we have lost all four engines, we are in a fall. We can use all the help you can offer.” They somehow managed to restart two of the engines, and made a rough landing at Anchorage International airport. No lives were lost – but the repairs to make that Boeing 747 flight-worthy again cost $80 million.
To put that in perspective, when I served as chief scientist for volcano hazards for the US Geological Survey, my entire science team’s annual budget was less than $20 million. There are over 1,600 volcanoes around the world.
There is another interesting GNSS application that you might find fascinating – I sure did. When Mount St Helens erupted on 1 October, 2004, we had just a week of accelerating racket on our seismic network beforehand for a warning. The extrusion was first seen on October 12 – and by pure luck I got the first photo of the new “spine” from a helicopter orbiting the steaming and fractured Crater Glacier. This dacite (63% – 68% silica) extrusion was 700 degrees C where it was coming up from the talus slope at its base. It came out like a tube of squeezed gray toothpaste. In fact, it resembled the back of a whale, so that became the name for the first of several domes that would be extruded: The Whale. It moved south through crumbling talus and ice until it hit the remaining south rim of the 1980 eruption. The geodesists wondered when it actually reached that wall – “When Did the Whale Hit the Wall?” A check of a GPS station on the other side, on the outside south slope of the volcano, answered the question. On November 17, 2004, that station suddenly started moving southward. Was it an effect of snow on the antenna? No, because the only direction it moved was south – by about 10 cm. The entire crater wall was shoved southward by about 4 inches by the growing dacite dome hitting it on the other side.
I’ll never forget the elation of scientists using GPS technology to answer a real question about an erupting volcano. But GNSS systems provide us more than just answers to our scientific curiosity.
In 2005 a sharp-eyed geodesist then based in Anchorage, Alaska, was routinely checking data from several GPS units installed on Augustine volcano in the middle of Cook Inlet, south of Anchorage. This had erupted in 1979 and nearly killed David Johnston, one of our brightest young geologists, who was then killed just a year later during the May 18, 1980 lateral blast, the opening eruption salvo of Mount St Helens.
In August 2005 our Anchorage-based geodesist noticed some differential movement between two GPS stations – the first subtle signal that inflation was starting – and notified the Alaska Volcano Observatory Scientist-in-Charge. A daily check-and-monitoring effort was started. Sure enough, the signal was real, showing above all the background noise – and it was continuing. Federal and State Emergency entities, along with the FAA (Federal Aviation Administration), were put on notice. In Late December the first VT’s – rock-breaking signals – started appearing on the seismometers. As they accelerated in frequency and amplitude, the USGS issued a warning: an eruption is imminent in hours or days. One day later, on January 16, 2006, Augustine erupted, and dusted Anchorage with ash. International flights were cancelled or re-routed for three days – but not a single aircraft was damaged, and not a single life was lost.
Yeah! This deformation stuff really works! And more to the point, it saves live.